Lab 10 - Electrochemical Cells
Purpose
To see how changes in concentration and pH affect the potential in an electrochemical cell, and confirm the Nernst equation.Goals
-
•To examine how standard reduction potentials are measured.
-
•To relate concentration changes to changes in cell potential.
Introduction
A galvanic cell is an electrochemical cell in which the spontaneous electrochemical reaction proceeds, that is, ΔG for the reaction is negative. The free energy decrease for a galvanic cell is proportional to the cell potential. The greater the driving force of the reaction, the greater the cell potential. The relationship is shown below.( 1 )
ΔG = −nFEcell
1
Identifying the oxidation (anode) and reduction (cathode) half-cells.
2
Looking up the standard half-cell potentials in a table of reduction potentials. An abbreviated table is included at the end of this lab procedure. Reminder: all potentials are listed in the table as reduction potentials.
3
Substituting the half-cell potentials into the following equation:
( 2 )
E°cell = E°cathode − E°anode.
( 3 )
Ecell = E°cell −
ln Q
RT |
nF |
ΔG = −nFEcell
and E°cell is the cell potential at standard conditions. Q is defined as the [products]coeff / [reactants]coeff. Similar to K, the equilibrium constant, Q, only includes terms in the aqueous or gaseous state. An example redox reaction and the reaction quotient are given below.
2 Ag+(aq) + Mn(s) ![]() | Q = [Mn2+]/[Ag+]2 |
( 4 )
Ecell = E°cell −
log Q.
0.0592 |
n |
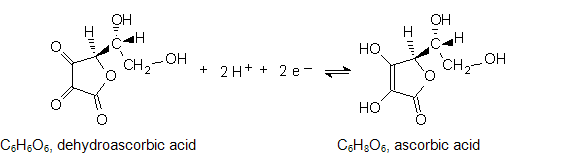
Figure 1
Equipment
Reagents
Safety
3 M HCl solution gives off highly irritating vapors. Do not inhale them. Work with concentrated solutions under the hood so vapors do not build up in the lab. If you do inhale enough vapor to have a problem, move to fresh air. Have your lab partner notify your instructor about the accident. HCl, NaOH, and KOH solutions are corrosive. They can attack the skin and cause permanent damage to the eyes. If the solution splashes into your eyes, use the eyewash immediately. Hold your eyes open and flush with water. If contact with skin or clothing occurs, flush the affected area with water. Have your lab partner notify your instructor about the spill. Cu2+, Pb2+, and Ag+ ions are listed as toxic. If contact with skin or clothing occurs, the affected area should immediately be flushed with water. If Ag+ solutions contact the skin, they will produce brown spots that appear about 24 hours after exposure. They are harmless and will fade in a few days.Waste Disposal
Test solutions that are provided in plastic containers in which measurements are to be made should not be discarded. When you are finished, close the containers with the proper lids and return them to the set-up area. You will rinse the salt bridge between measurements. The rinsings can be flushed down the sink. You should label a beaker as a rinse container and use it during the experiment, and empty it when you are finished. Diluted Cu2+ solution and any solid that forms in Part B should be put in the container labeled "Copper Waste." The ascorbic acid solution can be flushed down the sink with water.Prior to Class
Please read the following section of Lab Safety and Practices:Lab Procedure
Please print the worksheet for this lab. You will need this sheet to record your data.Part A: Measurement of Initial Cell Potentials
1
Open the MicroLab program. Make sure the alligator clip leads are connected to the interface.
2
Calibrate the mV probe as described in the MicroLab instructions provided in the lab. Note that the mV probe is factory calibrated, so you do not have to calibrate it yourself. However, to test the calibration of the mV probe, you can take a measurement with the leads connected to each other. This should give you a reading of ~0 mV.
3
Then configure the MicroLab program to collect data using the instructions provided in the lab. Record the mV value displayed on the screen for each measurement in your lab manual.
4
Label a 400 mL beaker as a rinse container.
5
Open the containers of Zn2+ and Ag+ solution. Put the lids where they will stay clean, and you can find them again.
6
Remove the salt bridge, with lids, from the containers of KNO3 solution.
7
If the solution in the salt bridge is low (not across the bridge) add KNO3 solution from the plastic container.
8
Rinse the salt bridge with deionized water (into the labeled waste beaker) and blot dry.
9
Immerse the salt bridge in the Zn2+ and Ag+ solutions, one leg in each, as shown in Figure 2.
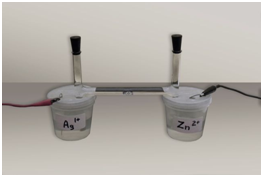
Figure 2: Electrochemical Cell Setup
10
Use the alligator clip on the black lead to pick up the zinc wire and place it in the Zn2+ solution. The wire should not be completely submerged.
11
Use the alligator clip on the red lead to pick up the silver wire and place it in the Ag+ solution. The wire should not be completely submerged.
12
Read the voltage. If the value is negative, reverse the leads. Record the voltage in Table A.
13
Reverse the leads on the metal wires. Measure the voltage and enter it in Table A.
14
Using the alligator clip, replace the silver wire in the labeled test tube.
15
Remove the salt bridge from the solutions. Rinse it with deionized water (into the labeled waste beaker) and blot dry.
16
Replace the lid on the Ag+ solution and set it aside.
17
Open the container of Pb2+ solution and put its lid where it will stay clean.
18
Immerse the salt bridge in the Zn2+ and Pb2+ solutions, one leg in each, as shown in Figure 2.
19
Replace the black lead on the Zn wire. Use the alligator clip on the red lead to pick up the lead wire and place it in the Pb2+ solution. The wire should not be completely submerged. Record the voltage in Table A.
20
Repeat the procedure for the Cu2+/Cu couple.
Part B: Dependence of Cell Potential on [Cu2+]
1
Combine an eyedropper full of 0.1 M Cu2+ solution with about 60 mL of deionized water in a 100 mL beaker.
2
Measure the potential of the diluted Cu2+ solution versus the Zn2+/Zn couple and record in Table B.
3
Add two eyedroppers full of 1.5 M KOH to the diluted Cu2+ solution, gently swirl the mixture, and observe the reaction.
4
Allow any solid that may have formed to settle to the bottom of the beaker, then measure the potential of the solution versus the Zn2+/Zn couple. Record this value in Table B.
5
Dispose of the diluted Cu2+ solution in the waste container located on the side shelf. Rinse with deionized water from your squirt bottle and add the rinsings to the waste bottle.
Part C: pH Dependence of Cell Potential
1
Prepare 60 mL of a solution that is 0.10 M in ascorbic acid (C6H8O6) and 0.10 M in sodium hydrogen phosphate (Na2HPO4 · 7 H2O). You can weigh both solids into the 100 mL beaker and add 60 mL of deionized water to it afterwards. Gentle swirling should dissolve the solids quickly.
2
Using a hand-held pH meter, adjust the pH of the solution to pH 6.8 - 7.2 by adding 3 M HCl or 3 M NaOH dropwise. It should not take more than 20 drops of either.
3
Assemble the electrochemical cell with the Cu2+/Cu couple as one half-cell and the buffered ascorbic acid solution with a graphite electrode as the other.
4
Measure the voltage. Make sure it is positive; if not, exchange the leads to the copper and graphite electrodes. Record the voltage and note which lead is connected to copper and which is connected to graphite in Table C.
5
Use a hand-held pH meter, and adjust the pH of the solution to pH 4.8 - 5.2. Use 3 M HCl added dropwise. 3 M NaOH is available if too much acid is added.
6
Reassemble the cell, with the leads in the same positions as they were in Step 4.
7
Measure and record the voltage in Table C.
8
When you are finished, rinse the salt bridge and return it to the KNO3 containers and close the lids. Replace the lids to any of the other solutions that are still open. Dispose of the waste rinsings and buffered ascorbic acid solution down the drain with plenty of water. Rinse all of your glassware with water, dry it and return it to the set-up area where you found it.
9
Please close the MicroLab program and turn off your pH meter.
10
Before leaving, enter your results in the in-lab assignment. If all results are scored as correct, log out. If not all results are correct, try to find the error or consult with your lab instructor. When all results are correct, note them and log out of WebAssign. The in-lab assignment must be completed by the end of the lab period. If additional time is required, please consult with your lab instructor.
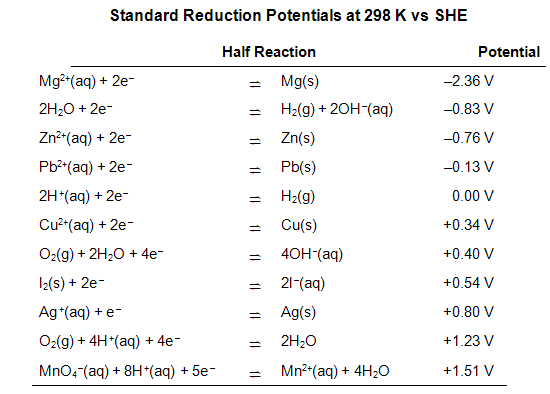